Will quantum theory and relativity meet at the nanoscale?
by Alan S. Brown
Research Highlights from Kavli Nanoscience Institutes
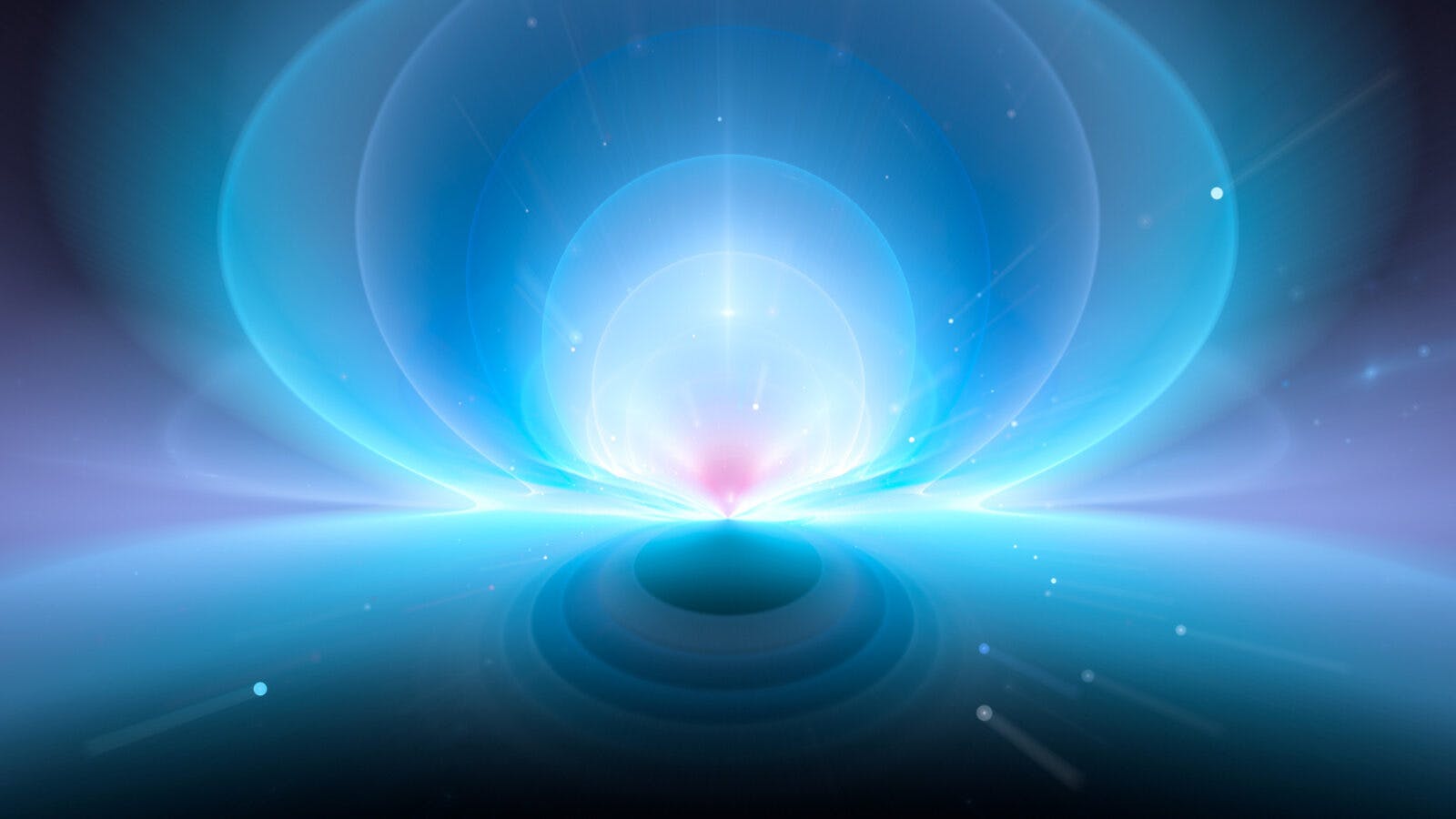
The Author
As the Kavli Nanoscience Institutes sprint ahead, big things are happening in biology and physics. Kavli researchers at Delft, for example, have developed a physics-based model of CRISPR-Cas9 that will give geneticists a much better idea of what DNA segments they are actually slicing. Caltech researchers have invented a way to colonize tumors with medicine-containing E. coli that will release their medication on command. Meanwhile, Cornell has licensed a technique to use DNA fragments in blood and urine to diagnose organ and tissue diseases and reduce or eliminate the need for painful biopsies. In the world of physics, Delft scientists have received a grant to use massive quantum objects to probe the incompatible theories of quantum mechanicals and general relativity. And finally, Cornell researchers have developed a thin nanocoating that enables nickel anodes to compete with far more expensive platinum anodes in alkaline fuel cells.
Will quantum theory and relativity meet at the nanoscale?
Gary Steele, a member of the Kavli Institute of Nanoscience at Delft Technical University, has received a grant of up to €1.5 million to study how quantum mechanics and gravity interact at the nanoscale. What makes these experiments unique is that gravity is governed by the theory of general relativity, which is fundamentally incompatible with quantum mechanics. Why? Because in relativity, mass changes the behavior of time; in quantum mechanics, mass can be in two places at the same time (called a superposition), which makes time uncertain (which it is not in relativity). To probe this disconnect, Steele and former graduate student Mario Gely (now at University of Oxford) have created a superconducting microelectromechanical system (MEMS) they can place in superposition. They hope the superpositioned mechanical structures have enough mass to push their quantum “clocks” out of sync, opening a door onto an unexplored realm in physics.
CRISPR model could reduce splicing errors
CRISPR-Cas9 took the gene editing world by storm because it makes it easy to target and edit specific segments of DNA. Unfortunately, it also splices untargeted (off-target) segments of DNA as well, which can lead to unforeseen consequences. Now, Martin Depken, a member of the Kavli Institute of Nanoscience at Delft Technical University, has developed a model to predict where and why targeting errors are most likely to occur. It differs from existing models, which predict only some strong off-targets and miss less likely interactions (whose total numbers may exceed their stronger cousins). This is because Depken’s model is based on the underlying physics of CRISPR-Cas9. He built the model from data gleaned with high-throughput experiments and single-molecule measurements. This enables Depken’s model to do something other models cannot: assign probabilities to possible splices and show how the odds change with time and CRISPR concentration. This could lead to better ways to assess risk and reduce off-target splicing.
Could E. coli help kill cancer?
Chemotherapy has saved or extended many lives, but it also kills healthy cells. That is why medical researchers are always looking for a better way to target tumors without affecting nearby tissue. Mikhail Shapiro, a member of the Kavli Nanoscience Institute at Caltech, has developed an elegant way to surmount this barrier. It starts with a strain of Escherichia coli (E. coli) bacteria modified to colonize cancer tumors. Ordinarily, the body destroys loose E. coli cells, but it cannot attack colonies once they have infiltrated the tumors. The E. coli are also modified to produce nanobodies that turn off the tumors’ immune system defenses once the E. coli reach a certain temperature. To get them to that temperature, Shapiro uses a technology he has been working on for years—focused ultrasound. By directing it at the tumors, he can warm the E. coli without damaging surrounding tissue. Once warmed, the E. coli release their nanobodies directly into the tumor. Research shows tumors in mice treated with bacterial and ultrasound grew far more slowly than those treated with bacteria alone or not treated at all.
Biopsy-free way to assess organ damage
Billions of DNA fragments course through our bloodstream and urine, and each of them tells a story. Over the past few years, Iwijn De Vlaminck, a member of the Kavli Institute at Cornell for Nanoscale Science, has developed ways to listen to those stories so he can trace diseases back to the organs and tissues from which they came. This is no small trick, since every cell in our bodies has the same DNA. De Vlaminck’s insight was to study how different types of cells and organs use the same DNA differently and focus on how that affected the DNA it gave off. His sampling technique reduces or entirely eliminates the need for painful biopsies. Cornell has licensed the technology to Eurofins Viracor, a company that specializes in testing for infectious disease. Viracor plans to use the technology to identify complications in patients infused with blood stem cells and urinary tract infections in kidney transplant patients.
Precious-metal-free fuel cells
Alkaline fuel cells powered the Apollo moon mission in 1969, yet they never went mainstream. That is because they require expensive catalysts made of platinum or other precious metals to withstand their alkaline environments. Attempts to create nonprecious metal catalysts have failed to achieve the necessary performance and durability. Now, Héctor D. Abruña, a member of the Kavli Institute at Cornell, has developed a nickel anode coated with a 2-nanometer-thick layer of nitrogen-doped carbon that promises to combine performance with low cost. The carbon layer prevents oxides from growing on the nickel surface and degrading reaction speed and catalyst lifespan. It also tolerates byproduct carbon monoxide gases, which rapidly poison platinum catalysts. When Abruña’s team paired the anode with a cobalt-manganese cathode to create a precious-metal-free hydrogen fuel cell, they achieved an energy density of 200 milliwatts per square centimeter—competitive with platinum-based systems.