Diving Deep
by Alan S. Brown
Research Highlights from Kavli Nanoscience Institutes
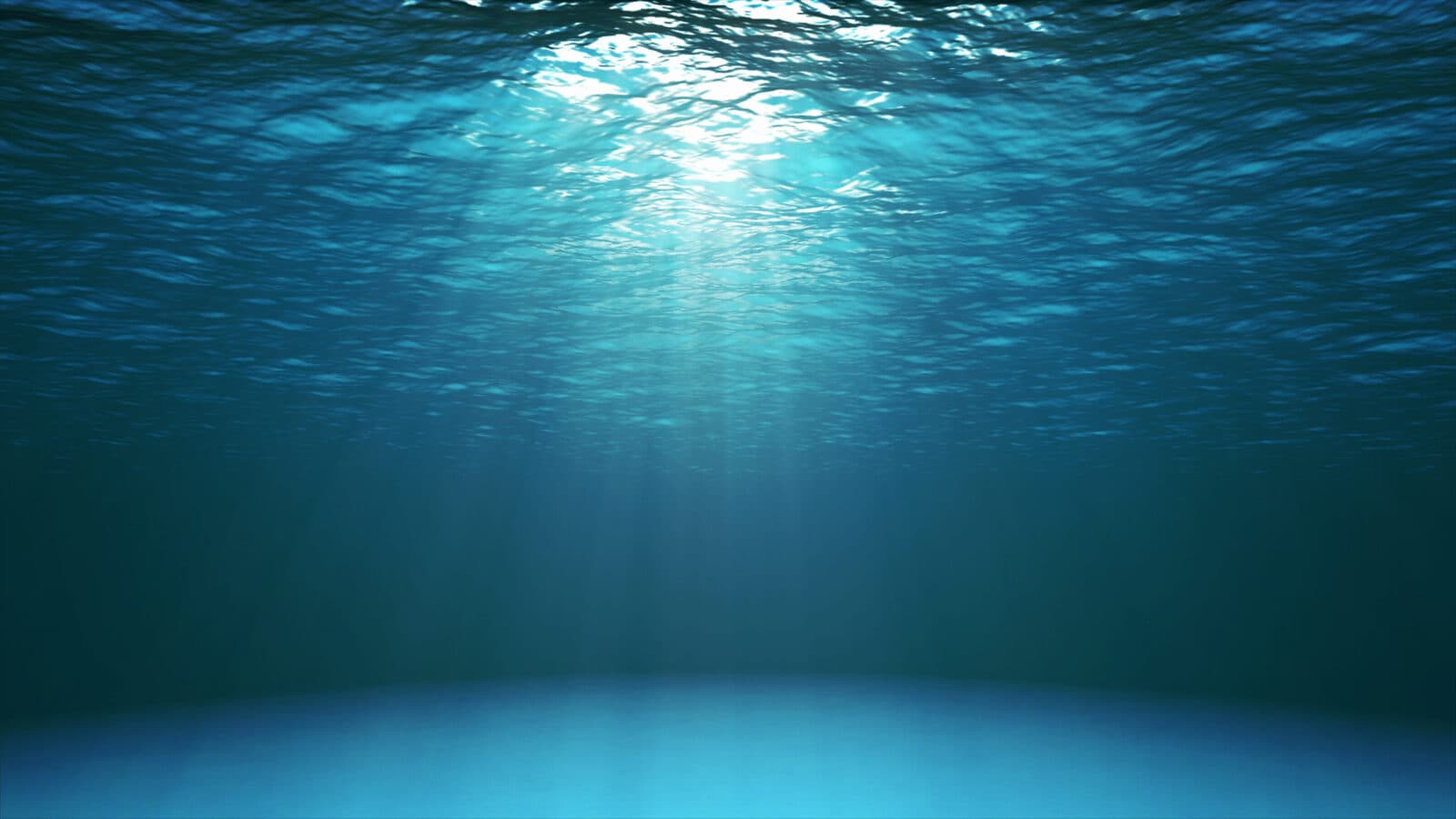
The Author
Because nanoscience dives into the detailed workings of our bodies and our world, the details and confusing nomenclature often obscure big breakthroughs. This month offers a fine example. It involves G-protein-coupled receptors (GPCRs), molecules that sit on a cell's surface and send signals across the cell membrane when stimulated. They play a key role in how our cells sense and react to the outside world. They determine how we sense light, taste, smell, and pain, as well as how our cells react to hormones, nerve, and other signals. Because of this, many medications target them. The trouble is, we don't really understand the type of reactions they trigger in cell membranes. Now, researchers at the Kavli Institute for Nanoscience Discovery at Oxford University have discovered a way to resolve these interactions, creating an entirely new set of targets for potential drugs. We will also look at how genetics shape our microbiome, how graphene drums can tell if bacteria are alive or dead, a better laser for disinfection, and an X-PRIZE milestone awardee that promises to remove massive amounts of carbon dioxide from the world's oceans.
Improved drug discovery
Many medications target G-protein-coupled receptors (GPCRs), which alert cells of outside stimuli, to manage everything from high blood pressure and enlarged prostates to ulcers, migraine headaches, and potentially, cancer. Yet researchers cannot test drug candidates on GPCRs in their native cell membranes. Instead, scientists encapsulate them in artificial detergent bubbles called micelles. Yet micelles do not truly simulate the membrane environment. To probe more deeply, Carol Robinson, director of Oxford University's Kavli Institute for Nanoscience Discovery, dragged a mass spectrometer into a dark room to monitor the real-time cascade of signals that occur when she exposed a membrane containing the GPCR receptor rhodopsin to light. She found that the reactions are significantly slower in the membrane than the micelles. She also demonstrated the importance of membrane lipids in signaling, coupling, effector targeting, and regeneration. By illuminating the full cascade of signals, she opens the door to more potential drug targets.
How our genes shape our microbiomes
Over the past decade, researchers have come to realize the extent to which out gut microbiomes – the trillions of bacteria, archaea, and fungi that inhabit our intestines – impact metabolism, health, and disease. What we don't know is how our genetic makeup impacts the makeup and functions of our microbiomes. That is the problem Kavli Institute at Cornell for Nanoscale Science researcher Ilana Brito and her collaborators in genetic variation and biostatistics hope to unravel. This is not an easy problem to solve. The human genome varies from person to person and even within paired chromosomes of the same person. To simplify the problem, Brito's team focused on variations caused by the substitution of a single nucleotide in DNA. Using novel computational and modeling approaches, the researchers then correlated those variations with several microbiome-associated disorders and cancers. They were able to show many instances where a person's genetic makeup directly affected how his or her microbiome performed.
Dead bacteria don't drum
How can you tell if a disease-causing bacterium is dead? This is actually a very practical question, since it is vital to diagnosing whether bacteria are resistant to a specific antibiotic. A new approach uses graphene to monitor the noise made by the biological processes of bacteria on its surface. Graphene has unusual electrical and mechanical properties and is extremely sensitive to external forces on its surface. In fact, it can even pick up the small movements of flagella, the small hairs that one-celled organisms use to propel themselves forward. When the flagella beat on the graphene surface, it acts like the head of a drum and produces a series of random oscillations that the researchers could detect. When antibiotics work, the number of oscillations decline and eventually cease. The technique was developed at Delft Technical University by Farbod Alijani and two members of the Kavli Institute of Nanoscience at Delft, Cees Dekker and Peter Steeneken.
Laser promises to kill pathogens
Deep-ultraviolet lasers (operating at of about 200-300nm wavelengths) can kill germs, sterilize medical instruments, purify water, and sense hazardous gases. The problem is creating affordable deep-UV lasers on chip-based diodes. At the Kavli Institute at Cornell, researchers led by Debdeep Jena, Huili Grace Xing, and their graduate student, Len van Deurzen, have shown they can produce a 284nm laser beam that achieves the frequencies and purity needed to meet the challenge. The material involved is aluminum gallium nitride (AlGaN). The researchers grew their AlGaN crystal by alternating layers of AlGaN with slightly different ratios of aluminum and gallium. By using a technique called molecular beam epitaxy, they were able to keep the interfaces between the layers very sharp, which contributed to the performance of the laser.

Removing carbon from water
When most of us hear "carbon removal," we think about atmospheric carbon dioxide. After all, carbon in the air causes the greenhouse effect that is heating the planet. To limit climate change to 1.5 degrees C, humanity will have to stop adding greenhouse gases to the atmosphere and start removing 10 gigatons of CO2 per year by 2050. To help find a way to do that, the XPRIZE Foundation is offering a total of $100 million in awards for scalable carbon removal technologies. This year, it picked 15 milestone projects with the potential to make a significant dent in carbon removal. While many focus on atmospheric carbon, one led by Harry Atwater of the Kavli Nanoscience Institute at Caltech and Chengxiang Xiang, seeks to remove carbon from seawater using a newly patented bipolar membrane. Ocean water is a surprisingly good target for carbon removal for two reasons. First it has absorbed 40 percent of all carbon emissions since the start of the industrial revolution. Second, its carbon concentration is 120 times greater than air. Removing carbon from ocean water would enable it to absorb even more CO2 from the atmosphere – and also reduce ocean acidification, which is destroying coral reefs and other marine habitats.